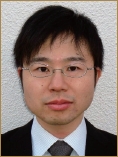
Low back pain is a global health problem with a markedly high lifetime prevalence— 70%–85% [
1]—and socioeconomic burden—up to $102.0 billion/yr in the United States [
2], which is also the most common reason for the worker’s disability [
3]. Although the cause of low back pain is largely nonspecific, a large-scale twin study has found intervertebral disc degeneration as the independent, main risk factor for disabling low back pain [
4]. A population study of magnetic resonance imaging has further identified the increase in the prevalence of lumbar disc degeneration with age, based on 42% of 18–30 years and 88% of 50–55 years in age [
5], thereby facilitating impaired daily activities of the elderly [
6]. Along with severe low back pain, intervertebral disc degeneration can cause neurological disorders such as radiculopathy, myelopathy, paralysis, intermittent claudication, and even bladder and bowel dysfunction [
7]. Despite successful conservative treatment for degenerative disc disease [
6], nonresponders need surgery [
7]. The current primary surgical approach is symptomatic disc excision and/or spinal fusion, which results in the loss of load bearing, shock absorption, and movement [
7]. Therefore, the development of new biological therapies for degenerative disc disease is an urgent demand to restore the physiological function.
The intervertebral disc is unique, as the largest immune-privileged, low-nutrient, avascular organ in the human body [
8]. The disc has a complex structure of the central nucleus pulposus (NP) encapsulated by the peripheral annulus fibrosus (AF) and sandwiching cartilage endplates [
7]. The collagenous, laminar AF maintains the pressurization of the gelatinous, oval NP, which provides support during compressive loading and multidimensional spinal movement [
9]. In the disc development, while the AF comes from the mesenchyme [
10], the NP originates from the notochord [
11]. In human life, disc NP notochordal cells only exist during the first 10 years [
10], later replaced by nonnotochordal, chondrocyte-like cells arising from NP cells as a terminal differentiation stage during degeneration [
12]. The phenotype of AF cells is thus chondrocytic while NP cells have the chondrocytic or notochordal phenotype depending on the age and degeneration severity [
13]. Accompanied with the notochordal phenotype disappearance and chondrocyte proliferation, cell death increases between 11–16 years of age in the disc [
14]. Under stress [
15], cells can fall into the irreversible growth arrest of senescence, producing proinflammatory cytokines and matrix-degrading proteases through the senescence-associated secretory phenotype [
16]. Excessive stress ultimately leads to programmed cell death including apoptosis, necroptosis, pyroptosis, and ferroptosis [
16]. Autophagy, the intracellular degradation and recycling system, also plays a role in maintaining cell homeostasis [
16,
17]. Taken together, cell fate with the phenotypic changes should be involved significantly in the pathogenesis of intervertebral disc degeneration.
Morphological disc degeneration also begins during early childhood [
14,
18], which is more severe in the NP than in the AF [
14]. Disc NP clefts and radial tears can appear between 11–16 years of age [
14]. Biochemically, disc cells have anabolic activities through the production of extracellular matrix components, e.g., proteoglycans—primarily aggrecan—and collagens—principally types I in the AF and II in the NP [
7]. Aggrecan biosynthesis and procollagen type-II content peak at ≤ 5 years and diminish between 5–15 years of age [
18]. Meanwhile, denatured collagen type II increases after the age of 5 years [
18]. This matrix degradation is regulated by the balance between catabolic enzymes, e.g., matrix metalloproteinases (MMPs) and a disintegrin and metalloproteinase with thrombospondin motifs (ADAMTSs), and anticatabolic proteins, e.g., tissue inhibitors of metalloproteinases (TIMPs) [
19]. In fact, increased MMP and ADAMTS activity relative to TIMP expression has been shown in human [
20,
21] and rodent experimental disc degeneration [
22]. Hence, extracellular matrix degradation is a key hallmark of intervertebral disc degeneration.
A narrative literature review article by Sao and Risbud [
23], provides the extensive, expertized summary of proteoglycans, a major component of the extracellular matrix. Proteoglycans consist of a core protein covalently attached with glycosaminoglycan chains. Proteoglycans serve for the hydration absorbing and retaining water in the tissue through their sulfated glycosaminoglycans, which are essential for the disc to support the trunk. Proteoglycans are categorized into the 4 groups based on the type of glycosaminoglycan chains: chondroitin sulfate/dermatan sulfate, heparan sulfate, keratan sulfate, and hyaluronic acid [
24]. These four types are further categorized depending on the size of proteoglycans: large and small [
24]. In the extracellular matrix, while large proteoglycans provide water-binding properties, tissue hydration, and growth factor bioavailability, small proteoglycans regulate collagen fibril formation [
24]. Recently, the categorization based on the location has been suggested: extracellular, pericellular, cell surface, and intracellular [
24]. Aggrecan is the most abundant, large extracellular proteoglycan with chondroitin sulfate/dermatan sulfate chains. Versican is another large extracellular proteoglycan with chondroitin sulfate chains. Lubricin has been shown as an atypical extracellular proteoglycan. Biglycan and decorin are small extracellular proteoglycans with chondroitin sulfate/dermatan sulfate chains. Fibromodulin and tenomodulin are also small proteoglycans present in the extracellular matrix, contributing to the tissue structural integrity. Syndecans, particularly syndecan-4, are a cell surface proteoglycan, which mediate multiple musculoskeletal diseases through inflammation, such as rheumatoid arthritis, osteoarthritis, and intervertebral disc degeneration [
25]. Perlecan is a large pericellular proteoglycan with heparan sulfate/chondroitin sulfate chains. This review article [
23] describes in detail not only intervertebral disc and cartilage pathologies but also skeletal dysplasias in humans and mouse models resulting from the loss or reduction of proteoglycans. Serious congenital abnormalities and embryonic lethality by the deletion of proteoglycans indicate their essentiality in skeletal development and integrity. In particular, the structure and function of major proteoglycans should be known, as spine surgeons, specialists, and researchers often manage discs and facet joints. Finally, as a conclusive remark [
23], the authors further propose potential biological therapies to treat disc degeneration and restore the matrix function, including senolytic approaches. This paper [
23], published in the March 2024 issue of the
Neurospine, provides the fundamental basis and important updates for a better understanding of intervertebral disc cell matrix biology.